Bio-RAD
Electroporation Systems
MicroPulser Operating Instructions and Applications Guide 165-2100 Rev B
Operating Instructions and Applications Guide
31 Pages
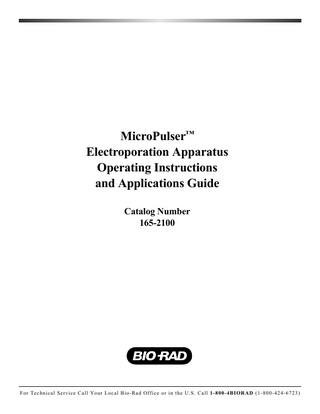
Preview
Page 1
MicroPulser™ Electroporation Apparatus Operating Instructions and Applications Guide Catalog Number 165-2100
For Technical Service Call Your Local Bio-Rad Office or in the U.S. Call 1-800-4BIORAD (1-800-424-6723)
Table of Contents Page
Section 1
Safety Information ...1
1.1 1.2 1.3
Electrical Hazards...1 Mechanical Hazards ...1 Other Safety Precautions...1
Section 2
Introduction ...2
2.1 2.2
Overview of electroporation theory ...2 Manipulation of instrument parameters...4
Section 3
Factors Affecting Electroporation ...5
3.1 3.2 3.3
Cell growth ...6 DNA...6 Electroporation Media...7
Section 4
MicroPulser Operating Instructions...9
4.1 4.2 4.3
Setting up the MicroPulser System...9 Operation of the MicroPulser...9 Electroporation using the MicroPulser ...11
Section 5
High Efficiency Electrotransformation of E. coli...12
5.1 5.2 5.3
Preparation of electrocompetent cells...12 Electroporation ...12 Solutions and reagents for electroporation ...13
Section 6
Electroporation of Staphylococcus aureus...13
6.1 6.2 6.3
Preparation of electrocompetent cells...13 Electroporation ...14 Solutions and reagents for electroporation ...14
Section 7
Electroporation of Agrobacterium tumefaciens ...15
7.1 7.2 7.3
Preparation of electrocompetent cells...15 Electroporation ...15 Solutions and reagents for electroporation ...16
Section 8
Electroporation of Saccharomyces cerevisiae...16
8.1 8.2 8.3
Preparation of electrocompetent cells...16 Electroporation ...16 Solutions and reagents for electroporation ...17
Section 9
Electroporation of Schizosaccharomyces pombe...17
9.1 9.2 9.3
Preparation of electrocompetent cells...17 Electroporation ...18 Solutions and reagents for electroporation ...18
Section 10
Electroporation of Dictyostelium discoideum ...18
10.1 10.2 10.3
Preparation of electrocompetent cells...18 Electroporation ...19 Solutions and reagents for electroporation ...19
Section 11
Electroporation of Pichia pastoris ...19
11.1 11.2 11.3
Preparation of electrocompetent cells...19 Electroporation ...20 Solutions and reagents for electroporation ...20
Appendix I
References ...21
Appendix II Troubleshooting Guide for the MicroPulser ...22 Appendix III Product Information ...25
Warranty Bio-Rad Laboratories warrants the MicroPulser against defects in materials and workmanship for 1 year. If any defects occur in the instrument during this warranty period, Bio-Rad Laboratories will, at Bio-Rad's option, repair or replace the defective parts free of charge. The following defects, however, are specifically excluded: 1. Defects caused by improper operation. 2. Repair or modification done by anyone other than Bio-Rad Laboratories or an authorized agent. 3. Use of fittings or other spare parts supplied by anyone other than Bio-Rad Laboratories. 4. Damage caused by accident or misuse. 5. Damage caused by disaster. 6. Corrosion due to use of improper solvent or sample. For any inquiry or request for repair service, contact Bio-Rad Laboratories after confirming the model, serial number, invoice number, and purchase order number of your instrument.
Model Catalog No. Date of Delivery Serial No. Invoice No. Purchase Order No.
Section 1 Safety Information Read This Information Carefully Before Using The MicroPulser. The MicroPulser meets the safety requirements of EN61010 and the EMC requirements of EN61326 (for Class B, including flicker and harmonics).
1.1 Electrical Hazards The MicroPulser produces voltages up to 3,000 volts and is capable of passing very high currents. When charged to maximum voltage, the instrument stores about 50 joules. A certain degree of respect is required for energy levels of this order. Safety system features prevent operator access to the recessed input jacks and to the recessed electrode contacts inside the sample chamber. These mechanical interlocks should never be circumvented. There is high voltage present whenever the yellow pulse button is depressed and "PLS" is shown in the light emitting diode display on the front of the instrument. If the capacitor has been partially charged but not fired (for example, when the charging cycle has been interrupted before the pulse is delivered), some charge may remain on the internal capacitor. However, the user cannot make contact due to the system safety features.
1.2 Mechanical Hazards The MicroPulser contains a patented arc-protection circuit that dramatically reduces the incidence of arcing in the cuvette when high voltage is delivered into the sample. The unit incorporates a circuit which senses the beginning of an arc and diverts current from the sample within ~5 µsec, preventing, or greatly reducing mechanical, visual, and auditory phenomena at the shocking chamber. Should an arc occur, the sample chamber is effective in containing these small discharges, but nonetheless we strongly recommend wearing safety glasses when using the instrument. Do not use the MicroPulser with samples suspended in conductive media (refer to Section 3.3 for information on sample resistance).
1.3 Other Safety Precautions Turn the unit off when not attended. Avoid spilling any liquids onto the apparatus. Use only a paper towel or a cloth wet with either water or alcohol to clean the outside surfaces of the MicroPulser. Use only the Bio-Rad cables supplied with the MicroPulser. Only use the shocking chamber in the assembled condition. Do not attempt to circumvent the protection of the shocking chamber or use it while disassembled. Verify the display segments periodically. Do not use the MicroPulser if obvious case damage exists that exposes part of the inside of the unit. No user-serviceable parts are contained within the MicroPulser; the case should only be opened by properly trained personnel.
1
Warning: The MicroPulser generates, uses, and radiates radio frequency energy. If it is not used in accordance with the instructions given in this manual, it may cause interference with radio communications. The MicroPulser has been tested and found to comply with the limits for Class A computing devices (pursuant to Subpart J of Part 15 of FCC Rules) which provide reasonable protection against such interference when operated in a commercial environment. Operation of this equipment in a residential area is likely to cause interference. In this case the user will be required, at their own expense, to take whatever measure may be required to correct the interference.
Section 2 Introduction 2.1 Overview of Electroporation Theory The MicroPulser system is used for the electroporation of bacteria, yeast, and other microorganisms where a high voltage electrical pulse is applied to a sample suspended in a small volume of high resistance media. The system consists of a pulse generator module, a shocking chamber, and a cuvette with incorporated electrodes (Figure 1). The sample is placed between the electrodes in the cuvette. The MicroPulser module contains a capacitor, which is charged to a high voltage; the module then discharges the current in the capacitor into the sample in the cuvette.
Fig. 1. MicroPulser consisting of Pulse Generator Module, Shocking Chamber and Cuvette.
2
The capacitance discharge circuit of the MicroPulser generates an electrical pulse with an exponential decay waveform (Figure 2). When the capacitor is discharged into the sample, the voltage across the electrodes rises rapidly to the peak voltage (also known as the initial voltage, V0), and declines over time, t, as follows, Vt = V0 [e -(t/τ)]
Equation 1
where τ = R x C, the time constant, a convenient expression of the pulse length. The resistance of the circuit, R, is expressed in ohms, and the capacitance of the apparatus, C, is expressed in microfarads. According to Equation 1, τ is the time over which the voltage declines to 1/e (~37%) of the peak value. The internal circuitry of the MicroPulser is designed to provide optimum electroporation of E. coli and S. cerevisiae, as well as many other microorganisms, in which the optimum transformation efficiency occurs at a time constant of approximately 5 msec. These electroporation conditions are achieved by using a 10 microfarad capacitor and by placing a 600 ohm resistor in parallel with the sample cuvette along with a 30 ohm resistor in series with the sample cuvette.
Voltage (V)
V0
V0 e
Time (msec)
Fig. 2. Exponential decay pulse from a capacitance discharge system. When the capacitor, charged to an initial voltage, Vo is discharged into cells, the voltage applied to the cells decreases over time so that at time t = τ, the voltage is (1/e) x Vo of the initial value.
In addition to the time constant, the electric field strength is the other instrument parameter that is important in determining transformation efficiency. The electric field strength, E, is the voltage applied between the electrodes and is described by E = V/d
Equation 2
where V is the voltage applied and d is the distance (cm) between the electrodes. The strength of the electric field and the size of the cells determine the voltage drop across each cell, and it is this voltage drop that may be the important manifestation of the voltage effect in electroporation.
3
The purpose of the 30 ohm series resistor in the MicroPulser is to protect the instrument circuitry should arcing occur. Under normal operation, when samples are in high resistance media, this resistor will not affect the voltage applied to the sample. However, this resistor will significantly decrease the voltage applied to the sample if the resistance of the sample is low. The fractional drop in voltage applied to the sample is given by R30 / (R30 + Rsample) When Rsample is 600 ohms, there is a 5% voltage drop to the sample [30 / (30 + 600) = 0.048]. For this reason, electroporation with the MicroPulser should not be performed in solutions with a resistance of less than ~600 ohms. This includes samples in which the growth medium was not adequately removed from the cells, DNA samples containing salt contributed by residual sodium chloride, or ligation mixtures. The MicroPulser is able to measure the resistance of the sample and will not pulse into very low resistance media.
2.2 Manipulation of Instrument Parameters Several parameters on the MicroPulser may be altered to achieve maximum transformation efficiency. These include the field strength, E, the time constant, τ, and the width of a truncated exponential decay pulse. The field strength may be manipulated in two ways. First, voltages between 200 and 3000 V may be set directly on the MicroPulser. This parameter is the most easily controlled. The process of varying the voltage while keeping all other conditions unchanged is the basis for most electroporation optimization procedures. Second, using cuvettes with different electrode gap widths permits a means of changing the field strength. For electroporation of microorganisms, 0.1 and 0.2 cm gap cuvettes are most often used. Electroporation of E. coli is generally carried out at a voltage of 1.8 kV (E = 18 kV/cm) when electroporating cells in 0.1 cm cuvettes and at a voltage of 2.5 kV (E = 12.5 kV/cm) when electroporating cells in 0.2 cm cuvettes. These electroporation conditions are pre-programmed into the MicroPulser as programs Ec1 (V = 1.8 kV) and Ec2 (V = 2.5 kV) in the bacterial settings menu. In addition, a third program, Ec3 in the bacterial settings menu, delivers a voltage of 3.0 kV (E = 15 kV/cm in 0.2 cm cuvettes) which we have found results in even higher transformation efficiency compared to electroporation at 2.5 kV. The time constant may be altered by changing the sample resistance. The sample resistance may be manipulated in two ways. First, increasing the salt or buffer concentration of the electroporation media decreases the resistance of the sample, and vice versa, resulting in a change in the time constant. Second, the volume of the sample in the cuvette is inversely proportional to the resistance of the sample; decreasing the sample volume increases the sample resistance. This effect of volume on sample resistance is most noticeable in low resistance media. These effects are discussed further in Section 3.3.
4
The MicroPulser also includes a means to truncate the exponential decay pulse sooner than the expected time constant as long as the voltage is greater than 600 V. When the pulse is terminated by the MicroPulser, voltage is applied to the sample only for the specified time, which may be between 1.0 and 4.0 msec. Figure 3 shows how this waveform differs from the true exponential decay pulse.
Voltage (V)
V0
V0 e
2.5
5 Time (msec)
Fig. 3. Truncation of an exponential decay pulse by the MicroPulser. The solid line shows the voltage applied to the cells as a function of time during a pulse terminated after 2.5 msec. The dashed line shows the voltage that would normally be applied to the cells during a true exponential decay pulse.
Section 3 Factors Affecting Electroporation The electrical conditions for the electroporation of microorganisms have been verified through years of research (see Chang, et al., 1992, and Nickoloff, 1995, for overviews as well as for protocols on electroporation of numerous species). For many microorganisms, optimum electrotransformation occurs under electrical conditions relatively similar to those used for E. coli and S. cerevisiae, two species that are most commonly used in research today. For electroporation of E. coli, conditions reported as being used most often are 0.2 cm cuvettes containing 40 µl of cells at a voltage of 2.5 kV and a time constant of ~5 msec. For electroporation of S. cerevisiae, conditions reported as being used most often are 0.2 cm cuvettes containing 40 µl of cells at a voltage of 1.5 kV and a time constant of ~5 msec. For many bacterial species, including Salmonella, Pseudomonas, Helicobacter, Borrelia, Streptococcus, Lactococcus, and Enterococcus, the conditions for electroporation are identical to those used for E. coli. For many other bacterial species, altering the field strength will often result in higher electrotransformation. A similar case is found with other species of yeast. The MicroPulser is designed to deliver precisely those pulse parameters needed for the highest transformation efficiency of E. coli and S. cerevisiae. The time constant has been set
5
at 5 milliseconds when working with high-resistance samples. For these organisms, the MicroPulser has pre-programmed settings for delivery of the correct voltage when electroporating E. coli in either 0.1 or 0.2 cm cuvettes, or when electroporating S. cerevisiae in either 0.2 or 0.4 cm cuvettes.
3.1 Cell Growth For most bacterial species, the highest transformation efficiencies are obtained when cells are harvested in early to mid-log growth. For E.coli, as the cells reach stationary phase, the transformation efficiency will decline precipitously (Dower, 1990). In contrast, most yeast species are generally harvested in mid- to late-log growth. For S. cerevisiae, the transformation efficiency increases as much as 60-fold from early to late-log cultures (Becker and Guarente, 1991). The optimal portion of the growth phase to harvest cells is generally dependent on the cell type. When preparing competent cells of a new species it is generally best to employ conditions worked out for use with the same genus. Suggestions for factors to consider and general methods for producing electrocompetent cells are discussed in the articles by Dower et al. (1992) and Trevors et al. (1992).
3.2 DNA While the majority of electroporation applications involve delivery of plasmid DNA to cells, it should be mentioned that nearly any type of molecule can be introduced into cells by electroporation, including RNA, proteins, carbohydrates, and small molecules. With few exceptions, when delivering autonomously replicating plasmids, the highest transformation efficiencies are obtained when electroporating supercoiled plasmid. However, electroporating plasmid that will integrate into the host genome is usually most efficient using linear plasmid. For example, Candida, Pichia, and Tetrahymena are transformed more efficently when transformed with linearized than with supercoiled integrating plasmids. In both E. coli and Listeria monocytogenes, the transformation efficiency of relaxed circular plasmid is only slightly lower than that of supercoiled plasmid (Leonardo and Sedivy, 1990, Park and Stewart, 1990). However, linear plasmid is about 103 - 104-fold less efficient than the corresponding circular plasmid in both E. coli and Streptococcus pyogenes (Shigekawa and Dower, 1988, Simon and Ferretti, 1991). Electroporation efficiency per mole of plasmid generally decreases as the plasmid size increases in numerous species, including E. coli (Leonardo and Sedivy, 1990, Siguret et al., 1994), Pseudomonas aeruginosa (Dennis and Sokol, 1995), and Streptococcus thermophilus (Somkuti and Steinberg, 1988). However, in some species, including Lactococcus lactis (Holo and Nes, 1995), Enterococcus faecalis (Cruz-Rodz and Gilmore, 1990), and Clostridium perfringens (Allen and Blaschek, 1990), transformation efficiency appears to be independent of plasmid size up to 20–30 kb. Although transformation of most microorganisms has been accomplished using plasmid DNA isolated by a variety of methods, the plasmid purity has an effect on transformation efficiency. Significantly lower transformation efficiencies are generated with unpurified miniprep plasmid DNA than with plasmid DNA purified by a variety of procedures. Plasmid produced using the Bio-Rad Quantum matrix is as efficient as CsCl-purified plasmid for transformation of microorganisms. Generally, for all types of microorganisms, the frequency of transformation increases with inceasing DNA concentration in the electroporation buffer. For E. coli, the frequency of transformation (transformants/survivor) is dependent on DNA concentration over at least six orders of magnitude (10 pg/ml to 7.5 µg/ml); within this range the DNA concentration determines the probablility that a cell will be transformed. At the higher DNA concentrations, up to 80% of the survivors are transformed (Dower et al., 1988). Because the number of
6
transformants recovered is the product of the transformation frequency and the number of cells present, the transformation efficiency (transformants/µg DNA) increases with cell concentration over the range of 109 to at least 3 x 1010 cells/ml. Therefore, to obtain a high transformation frequency, use high DNA concentration. To obtain high transformation efficiency, use high cell concentration (and low DNA concentration to avoid cotransformations). In each case, a small sample volume (20–50 µl) allows economical use of DNA and cells (see Dower et al., 1988, for a detailed discussion of these factors).
3.3 Electroporation Media The MicroPulser is designed for use with samples in high resistance media (>600 ohms). For this reason, when preparing electrocompetent cells, it is important to wash cells thoroughly to remove all traces of growth media. Failure to thoroughly remove the growth media from the cells may result in the sample arcing during electroporation. Cells should be washed at least three times with water or with non-ionic solutions, such as glucose, glycerol, sucrose, sorbitol, or polyethylene glycol. For many microorganisms, glycerol is a convenient electroporation medium, since it is recommended as a cryoprotectant for storage of cell cultures.
7
Fig. 4. Resistance of solutions of (A) NaCl and MgCl2 and of (B) buffers of NaPO4 at pH 6.1 and 7.3 and HEPES at pH 7.5. Resistance was measured in 0.2 cm cuvettes containing either 40 µl or 200 µl of solution at room temperature.
Figures 4A and B show the effect of concentration of several biologically important ionic solutions on sample resistance. Note that: (1) volume has a significant effect on sample resistance-for ionic solutions, sample resistance is inversely proportional to the volume of solution in the cuvette; (2) the resistance of a solution containing divalent ions is lower than a solution containing the same concentration of monovalent ions; (3) the resistance of a buffered solution is affected by its pH. The addition of even small concentrations of ionic compounds significantly reduces the resistance of the sample and may cause arcing. Residual salt from ethanol precipitation of DNA should be reduced by washing the DNA pellet prior to dissolving it in either water or Tris-EDTA. Table 1 shows that, although adding a solution of plasmid in 10 mM Tris, pH 8.0–1 mM EDTA to water does reduce the sample resistance, this should not result in the inability to electroporate a sample in the MicroPulser. DNA may be used directly from enzyme reactions for transformation, but the final salt concentration in the electroporation sample should be kept below ~5 meq for high voltage operation. Finally, ligation mixtures may be used for transformation, but only in very low quantities or when the ionic strength is reduced by dilution (Willson and Gough, 1988), dialysis (Heery and Dunican, 1989; Jacobs et al., 1990), or ethanol precipitation (Böttger, 1988; Zabarovsky and Winberg, 1990).
Table 1. Resistance of Water in 0.2 cm Cuvettes To Which TE Has Been Added1. SAMPLE
Rsample (40 µl volume)
Rsample (200 µl volume)
Water
> 6 x 105
> 6 x 105
Water + 1 µl TE
> 6 x 105
35,000
Water + 5 µ TE
11,200
8,700
Water + 10 µl TE
4,850
4,700
1 The resistance of 0.2 cm cuvettes containing either 40 or 200 µl water and the indicated
volume of TE (10 mM Tris, pH 8.0, 1 mM EDTA) was measured at 1000 V.
8
Fig. 5. MicroPulser control panel.
Section 4 MicroPulser Operating Instructions Refer to Figure 1 for a view of the components of the MicroPulser system and to Figure 5 for a definition of the buttons and LEDs.
4.1 Setting Up The MicroPulser System 1. Connect the black power cord to the rear panel of the MicroPulser Pulse Generator Module. Plug the cord into a wall outlet or power strip. 2. Pull down the fold-down foot on the underside of the MicroPulser. Insert this foot into the track on the base of the shocking chamber. Insert the shocking chamber slide into the shocking chamber. 3. Connect the leads from the shocking chamber to the output jacks on the front panel of the MicroPulser; polarity is not important to the electroporation process. 4. Turn on the apparatus using the power switch on the right rear panel. The light emitting diode (LED) display should illuminate and read “Ec1” and the LED next to the Bacteria Settings should be illuminated.
4.2 Operation of the MicroPulser 1. Selecting Pre-Programmed Settings The MicroPulser is pre-programmed with settings for electroporation of a number of commonly used organisms. Included under the Bacteria Settings program are the following: Mnemonic
Ec1 Ec2
Organism Voltage (kV) 1.8 2.5
E. coli (0.1 cm cuvette) E. coli (0.2 cm cuvette)
9
Parameters Number of pulses 1 1
Time constant (msec) -
StA Agr Ec3
S. aureus (0,2 cm cuvette) A. tumefaciens (0.1 cm cuvette) E. coli (0.2 cm cuvette)
1.8 2.2 3.0
1 1 1
2.5 -
Included under the Fungi Settings program are the following: Mnemonic
Sc2 Sc4 ShS dic Pic
Organism
S. cerevisiae (0.2 cm cuvette) S. cerevisiae (0.2 cm cuvette) S. pombe (0.2 cm cuvette) D. discoideum (0.4 cm cuvette) P. pastoris (0.2 cm cuvette)
Voltage (kV) 1.5 3.0 2.0 1.0 2.0
Parameters Number of pulses 1 1 1 2 1
Time constant (msec) 1.0 -
Pressing the "Settings" button cycles the Settings LED between "Bacteria", "Fungi", and "Manual". When the LED next to Fungi is lit, the mnemonic for the fungi programs are displayed. Pressing the "Raise" and "Lower" buttons to the left of the display LED cycles between the different fungi programs. When the mnemonic is displayed, the parameters associated with the mnemonic are automatically selected. To change from one bacteria setting to another, while the Settings LED next to Bacteria is lit, press the "Raise" and "Lower" buttons to the left of the display LED to cycle between the different programs. When the mnemonic is displayed, the parameters associated with the mnemonic are automatically selected. While a program mnemonic is displayed for either a Bacteria or Fungi Setting, simultaneously pressing both the "Raise" and "Lower" buttons shows the program parameters selected on the display LED. The display LED first shows the voltage value, then displays a "t" followed by the time in msec, then, if time and multiple pulses are associated with a program, display "P" followed by "2", indicating that two successive pulses are given. If no "t" is given the pulse is not truncated, and if no "P" is given there is a single pulse. 2. Using The Micropulser in Manual Mode A. To change the voltage. Press the "Settings" button to illuminate the LED next to "Manual". The display LED now shows the voltage (in kV). Pressing the "Raise" and "Lower" buttons to the left of the display LED allows selection of the desired voltage in the range from 0.20 kV to 3.00 kV. If the instrument was just turned on, the display LED shows "0.00" B. To truncate the pulse. Press both "Raise" and "Lower" buttons simultaneously while the "Manual" LED is illuminated. The display LED now shows “t-” and indicates the pulse time that has been selected for the pulse. The default setting when the power is turned on is the standard exponential decay pulse, or no pulse truncation, which is indicated by the two dashes. Releasing only the "Lower" button results in the display LED showing the time of the truncated pulse in milliseconds. The display LED initially changes to "t1.0" and rises in 0.1 msec increments to "t4.0". This permits truncating the exponential decay pulse between 1–4 msec. Simultaneously pressing both "Raise" and "Lower" buttons, then releasing only the "Raise" button, results in lowering the indicated truncation time.
10
3. Pulse Function Pressing the "Pulse" button results in the capacitor charging to the set voltage; during this time "PLS" is shown on the display LED. A tone will sound to indicate that the pulse has been delivered. When multiple pulses are delivered by one of the built-in programs, "PLS" is shown on the display LED during the entire time and a tone sounds each time a pulse is delivered. To manually deliver multiple pulses, after the tone sounds from the first pulse, press the pulse button again. If a lower pitched tone sounds, accompanied by "Arc" being shown on the display LED, the arc prevention and quenching (ARQ) system has been actuated and the pulse has been terminated. This is usually an indication of attemped cuvette arc-over, but may also occur if the sample resistance is too low. Since the energy delivered during such an ARQ event is low, it is usually possible to pulse the sample again at parameters which will not result in an arc and still produce acceptable results. However, it is not advisable to use a sample in which two arc events have occurred. 4. Measurements Pressing the "Measurements" button results in illumination of the "Actual kV" LED. This indicates that the display LED shows the actual voltage delivered (in kV) during the last pulse. If the instrument was just turned on and no pulse has been given, the display LED shows "0.00". Pressing the "Measurements" button again results in illumination of the "Time ms" LED. This indicates that the display LED shows the time constant (in msec) of the last pulse. If the instrument was just turned on and no pulse has been given, the display LED shows "0.00". Holding the "Measurements" button toggles the display LED between the Actual voltage and the Time constant.
4.3 Electroporation Using The MicroPulser 1. Place the cell suspension in an electroporation cuvette and tap the liquid to the bottom of the cuvette. Up to 0.4 ml (400 µl) of solution may be placed in the 0.2 cm cuvette, and up to 80 µl may be placed in the 0.1 cm cuvette. Note that temperature may have a significant influence on transformation frequency. Electroporation of some organisms, including E. coli and S. cerevisiae, is more efficient in chilled cuvettes. 2. Insert the cuvette into the slide of the shocking chamber. Push the slide into the chamber until the cuvette makes firm contact with the chamber electrodes. 3. To charge the capacitor and deliver a pulse, press the yellow "Pulse" button; the display LED will show "PLS" until a tone sounds indicating that the pulse has been given. The display LED will then show the program, the time constant, or the actual volts delivered, depending on the LED selected. 4. Withdraw the slide from the chamber, remove the cuvette, and process the sample. 5. The time constant and the actual voltage delivered to the sample are shown on the display LED by pressing the "Measurements" button. When the LED next to "Actual kV" is illuminated, the voltage is displayed in kilovolts. The time constant can be displayed by pressing the "Measurements" button again. The LED next to "Time ms" will be illuminated; the time constant is displayed in milliseconds. 6. To turn the unit off press the power switch on the right rear panel. The sample chamber may now be safely disconnected, if desired. Never remove the sample chamber cover until the leads are disconnected.
11
Section 5 High Efficiency Electrotransformation of E. coli Electroporation provides a method of transforming E. coli at efficiencies as high as 109 to 1010 transformants/µg, which is greater than is possible with the best chemical methods. The following protocol describes a method for for preparing and electrotransforming E. coli to high efficiencies. We are interested in hearing of additional strains transformed by electroporation and including this information in subsequent versions in our Electroprotocols manual. Please contact your local Bio-Rad representative, access our web site at www.bio-rad.com, or, in the U.S., call our Technical Services at (800) 424-6723 with any comments or questions.
5.1 Preparation of Electrocompetent Cells See Ausubel et al. (1987) and Miller and Nickoloff (1995) for additional information. 1. Inoculate 500 ml of L-broth with 1/100 volume of a fresh overnight E. coli culture. 2. Grow the cells at 37 °C shaking at 300 rpm to an OD600 of approximately 0.5–0.7 (the best results are obtained with cells that are harvested at early- to mid-log phase; the appropriate cell density therefore depends on the strain and growth conditions). 3. Chill cells on ice for ~20 min. For all subsequent steps, keep the cells as close to 0 °C as possible (in an ice/water bath) and chill all containers in ice before adding cells. To harvest, transfer the cells to a cold centrifuge bottle and spin at 4000 x g for 15 minutes at 4 °C. 4. Carefully pour off and discard the supernatant. It is better to sacrifice the yield by pouring off a few cells than to leave any supernatant behind. 5. Gently resuspend the pellet in 500 ml of ice-cold 10% glycerol. Centrifuge at 4000 x g for 15 minutes at 4 °C; carefully pour off and discard the supernatant. 6. Resuspend the pellet in 250 ml of ice-cold 10% glycerol. Centrifuge at 4000 x g for 15 minutes at 4 °C; carefully pour off and discard the supernatant. 7. Resuspend the pellet in ~20 ml of ice-cold 10% glycerol. Transfer to a 30 ml sterile Oakridge tube. Centrifuge at 4000 x g for 15 minutes at 4 °C; carefully pour off and discard the supernatant. 8. Resuspend the cell pellet in a final volume of 1–2 ml of ice-cold 10% glycerol. The cell concentration should be about 1–3 x 1010 cells/ml. This suspension may be frozen in aliquots on dry ice and stored at -70 °C. The cells are stable for at least 6 months under these conditions.
5.2 Electroporation 1. Thaw the cells on ice. For each sample to be electroporated, place a 1.5 ml microfuge tube and either a 0.1 or 0.2 cm electroporation cuvette on ice. 2. In a cold, 1.5 ml polypropylene microfuge tube, mix 40 µl of the cell suspension with 1 to 2 µl of DNA (DNA should be in a low ionic strength buffer such as TE). Mix well and incubate on ice for ~1 minute. (Note: it is best to mix the plasmids and cells in a microfuge tube since the narrow gap of the cuvettes prevents uniform mixing.) 3. Set the MicroPulser to “Ec1” when using the 0.1 cm cuvettes. Set it to "Ec2" or "Ec3" when using the 0.2 cm cuvettes. See Section 4 for operating instructions.
12
4. Transfer the mixture of cells and DNA to a cold electroporation cuvette and tap the suspension to the bottom. Place the cuvette in the chamber slide. Push the slide into the chamber until the cuvette is seated between the contacts in the base of the chamber. Pulse once. 5. Remove the cuvette from the chamber and immediately add 1 ml of SOC medium to the cuvette. Quickly but gently resuspend the cells with a Pasteur pipette. (The period between applying the pulse and transferring the cells to outgrowth medium is crucial for recovering E. coli transformants (Dower et al., 1988). Delaying this transfer by even 1 minute causes a 3-fold drop in transformation. This decline continues to a 20-fold drop by 10 minutes. 6. Transfer the cell suspension to a 17 x 100 mm polypropylene tube and incubate at 37 °C for 1 hour, shaking at 225 rpm. 7. Check and record the pulse parameters. The time constant should be close to 5 milliseconds. The field strength can be calculated as actual volts (kV) / cuvette gap (cm). 8. Plate on selective medium.
5.3 Solutions and Reagents For Electroporation 1. L-Broth: 10 g Bacto tryptone, 5 g Bacto yeast extract, 5 g NaCl; dissolve in 1.0 L water. Autoclave. 2. 10% (v/v) Glycerol: 12.6 g glycerol (density = 1.26 g/cc) in 90 ml of water. Autoclave or filter sterilize. 3. TE: 10 mM Tris-HCl pH 8.0, 1 mM EDTA. 4. SOC: 2% Bacto tryptone, 0.5% Bacto yeast extract, 10 mM NaCl, 2.5 mM KCl, 10 mM MgCl2, 10 mM MgSO4, 20 mM glucose.
Section 6 Electroporation of Staphylococcus aureus 6.1 Preparation of Electrocompetent Cells See Lee (1995) for additional information. 1. Inoculate 3 ml of B2 broth in a 17 x 100 mm tube with a colony from a fresh S. aureus plate. 2. Incubate at 37 °C overnight, shaking at 250 rpm. 3. Inoculate 1.5 ml of the overnight culture into 150 ml of fresh B2 broth in a 1 liter flask. Incubate at 37 °C, shaking at 250 rpm, to ~2 x 108 cells/ml. The doubling time of S. aureus is about 30 min at 37 °C. 4. Chill the cells in an ice water bath for 15 min to stop growth. Decant the cells into a sterile 500 ml centrifuge bottle. Harvest the cells by centrifugation at 12,000 x g for 15 min at 4 °C. 5. Carefully pipette off the supernatant, keeping the cell pellet on ice. 6. Resuspend the cell pellet in 500 ml of sterile, ice-cold water. Pellet the cells by centrifugation at 12,000 x g for 15 min at 4 °C; carefully remove the supernatant. Wash the cells 2 more times in 500 ml of sterile, ice-cold water.
13
7. Resuspend the cell pellet in 25 ml of sterile, ice-cold 10% glycerol. Transfer to a 30 ml sterile Oakridge tube. Pellet the cells by centrifugation at 12,000 x g for 15 min at 4 °C; carefully remove the supernatant.. 8. Resuspend the cell pellet in 2 ml of 10% glycerol; the final cell concentration should be ~1 x 1010 cells/ml. 9. Dispense 250 µl aliquots of the electrocompetent cells into sterile 1.5 ml microfuge tubes; freeze the cells in an isopropanol-dry ice bath, then store at -70 °C. The cells are stable for several months under these conditions.
6.2 Electroporation 1. Pipette the DNA samples (5 ng - 2 µg in a volume ~ 3 µl) to be electroporated into sterile 1.5 ml microfuge tubes. 2. Thaw the competent cells at room temperature for several minutes. Add 50 µl of cells to each DNA sample; gently pipette up and down to mix. 3. Incubate the samples at room temperature for 30 min. 4. Set the MicroPulser to "StA". See Section 4 for operating instructions. 5. Transfer the mixture of cells and DNA to a 0.2 cm electroporation cuvette and tap the suspension to the bottom of the tube. Place the cuvette in the chamber slide. Push the slide into the chamber until the cuvette is seated between the contacts in the base of the chamber. 6. Pulse once. 7. Remove the cuvette from the chamber and immediately add 1 ml of SMMP medium containing a subinhibitory concentration of antibiotic; gently transfer the cells to a sterile 17 x 100 mm tube using a Pasteur pipette. Incubate 1 hr at 37 °C, shaking at 250 rpm. 8. Check and record the pulse parameters. The time constant should be close to 2.5 milliseconds. The field strength can be calculated as actual volts (kV) / cuvette gap (cm). 9. Plate aliquots of the electroporated cells on trypticase soy agar containing selective antibiotic. Incubate plates for 36–48 hrs at 37 °C.
6.3 Solutions and Reagents For Electroporation 1. B2 medium: 10 g casein hydrolysate, 25 g yeast extract, 5 g glucose, 25 g NaCl, 1 g K2HPO4; dissolve in 900 ml water and adjust pH to 7.5; bring volume to 1.0 L. Autoclave 2. SMMP: 55 ml 2X SMM, 40 ml 4X Penassay broth, 5 ml 10% (w/v) bovine albumin; adjust pH to 7.0; filter sterilize 3. Trypticase soy agar: 40 g trypticase soy agar (Becton Dickinson, Sparks, MD) in 1 L of water. Autoclave. 4. 2X SMM: 25 ml 0.2 M sodium hydrogen maleate, 40 ml 0.1 N NaOH; adjust the pH to 6.5. Add 5 ml 1 M MgCl2, 42.7 g sucrose; dissolve and bring volume to 125 ml. Filter sterilize. 5. 4X Penassay broth: 17.5 g Antibiotic Medium 3 (Becton Dickinson) dissolved in 250 ml water. Autoclave. 6. 0.2 M sodium hydrogen maleate: 11.6 g maleic anhydride or 13.7 g maleic acid, 4 g NaOH; dissolve in 500 ml water. Autoclave.
14
Section 7 Electroporation of Agrobacterium tumefaciens 7 .1 Preparation of Electrocompetent Cells See Lin (1995) for additional information. 1. Inoculate 1.5 L of YM broth in a 2.8 L Fernbach flask with an aliquot from log phase culture of A. tumefaciens. 2. Incubate at 30°C overnight, shaking at 300 rpm to a density of 5–10 x 107 cells/ml. 3. Decant the cells into sterile 500 ml centrifuge bottles and pellet the cells by centrifugation at 3000 x g for 10 min at 4 °C. 4. Carefully pour off and discard the supernatant; place the centrifuge bottles with the cell pellets on ice. 5. Add ~50 ml of sterile, ice-cold 10% glycerol to each of the bottles and vortex to resuspend the cell pellets; bring the volume in each of the centrifuge bottles to 500 ml with sterile, ice-cold 10% glycerol. Pellet the cells by centrifugation at 3000 x g for 10 min at 4 °C; pour off and discard the supernatant. 6. Wash the cells again as in step 5. 7. Resuspend each of the cell pellets in 5 ml of sterile, ice-cold 10% glycerol and transfer to a chilled 30 ml Oakridge tube. Pellet the cells by centrifugation at 3000 x g for 5 min at 4 °C; pour off and discard the supernatant. 8. Resuspend the cell pellet in 0.5 ml of sterile, ice-cold 1 M sorbitol; the final cell volume should be ~1.5 ml and the cell concentration should be ~ 5 x 1010 cells/ml. Dispense 200 µl aliquots of the electrocompetent cells in sterile 1.5 ml microfuge tubes; freeze the cells in an isopropanol-dry ice bath, then store at -70 °C. The cells are stable for about 6 months under these conditions.
7.2 Electroporation 1. Pipette the DNA samples (up to 5 µl) to be electroporated into sterile 1.5 ml microfuge tubes; the DNA should be in either water or TE. Place tubes on ice. 2. For each DNA sample to be electroporated, add 1 ml of YM broth to a 17 x 100 tube at room temperature, and place a 0.1 cm electroporation cuvette on ice. 3. Thaw the electrocompetent A. tumefaciens cells on ice. For each DNA sample to be electroporated, add 20 µl of electrocompetent cells to each DNA sample; gently tap the tubes to mix. 4. Set the MicroPulser to "Agr". See Section 4 for operating instructions. 5. Transfer the DNA-cell samples to the electroporation cuvettes and tap the suspension to the bottom of the tube. Place the cuvette in the chamber slide. Push the slide into the chamber until the cuvette is seated between the contacts in the base of the chamber. Pulse once. 6. Remove the cuvette from the chamber and immediately use the YM broth in the 17 x 100 mm tube to transfer the cells from the cuvette to the tube. 7. Check and record the pulse parameters. The time constant should be about 5 milliseconds. The field strength can be calculated as actual volts (kV) / cuvette gap (cm).
15
8. Incubate the cells 3 hr at 30 °C, shaking at 250 rpm. Plate aliquots of the electroporated cells on YM agar plates containing the appropriate selective media. Incubate plates for 48 hrs at 30 °C.
7.3 Solutions and Reagents For Electroporation 1. YM broth: 0.4 g yeast extract, 10 g mannitol, 0.1 g NaCl, 0.1 g MgSO4, 0.5 g K2HPO4.3H20, dissolve in 1.0 L of water and adjust to pH 7.0. Autoclave. For YM plates, add 15 g agar/1 L of YM broth.
Section 8 Electroporation of Saccharomyces cerevisciae 8.1 Preparation of Electrocompetent Cells See Becker & Guarantee (1991) and Ausubel et al. (1987) for additional information. 1. Inoculate 500 ml of YPD in a 2.8 L Fernbach flask with an aliquot from an overnight culture of S. cerevisiae. The doubling time of S. cerevisiae is approximately 2 hrs at 30 °C. 2. Incubate at 30 °C overnight, shaking at 250 rpm, to a density of ~1 x 108 cells/ml. 3. Chill the cells in an ice water bath for 15 min to stop growth. 4. Decant the cells into two sterile 250 ml centrifuge bottles and pellet the cells by centrifugation at 3000 x g for 5 min at 4 °C. 5. Carefully pour off and discard the supernatant; place the centrifuge bottles with the cell pellets on ice. 6. Add ~50 ml of sterile, ice-cold water to each of the bottles and vortex to resuspend the cell pellets; bring the volume in each of the centrifuge bottles to 250 ml. Pellet the cells by centrifugation at 3000 x g for 5 min at 4 °C; pour off and discard the supernatant. 7. Wash the cells again as in step 6 with a total of 250 ml sterile, ice-cold water. 8. Resuspend the cell pellet in 20 ml of sterile, ice-cold 1 M sorbitol and transfer to a chilled 30 ml Oakridge tube. Pellet the cells by centrifugation at 3000 x g for 5 min at 4 °C; pour off and discard the supernatant. 9. Resuspend the cell pellet in 0.5 ml of sterile, ice-cold 1 M sorbitol; the final cell volume should be ~1.3 ml and the cell concentration should be ~1 x 1010 cells/ml. Keep the cells on ice and use as soon as possible for electroporation.
8.2 Electroporation 1. Pipette the DNA samples (5–100 ng in a volume of 5 µl) to be electroporated into sterile 1.5 ml microfuge tubes. Place tubes on ice. 2. If 0.2 cm cuvettes are used, add 40 µl of the competent cells to each DNA sample; if 0.4 cm cuvettes are used, add 80 µl of the competent cells to each DNA sample. Mix gently and incubate on ice for ~5 min. 3. Set the MicroPulser to “Sc2” when using 0.2 cm cuvettes or to “Sc4” when using 0.4 cm cuvettes. See Section 4 for operating instructions.
16