Siemens
MR Spectroscopy Version syngo MR 2002B Operators Manual Rev 04 July 2002
Operators Manual
488 Pages
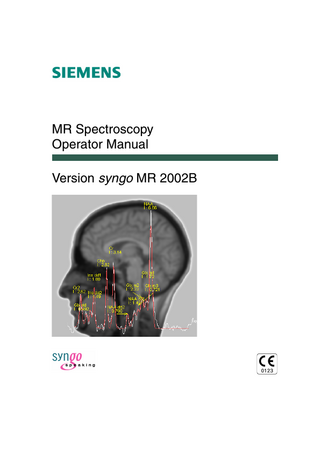
Preview
Page 1
MR Spectroscopy Operator Manual Version syngo MR 2002B
0.0
Manufacturer’s note:
0.0
Products that bear a CE mark fulfill the provisions of the Council Directive 93/42/EEC of 14 June 1993 regarding medical equipment. 0.0 The CE mark applies exclusively to medical equipment and products that are released under the relevant EU guidelines mentioned above. 0.0 0.0
0.0
0.0
0.0
0.0
0.0
0.0
0.0
0.0
0.0
0.0
0.0
0.0
0.0
0.0
Siemens AG 2002 All Rights Reserved
0.0
Siemens AG, Medical Engineering, Magnetic Resonance Henkestraße 127, D-91052 Erlangen, Germany
0.0
Headquarters in Berlin and Munich
0.0
Siemens AG, Wittelsbacher Platz 2, D-80333 München, Germany
0.0
Print-Nr. M1-015.630.02.01.02 Printed in the Federal Republic of Germany AG 07.02
0.0
0.0
MR Spectroscopy
Licenses
R.0
The following table lists the MR spectroscopy measurement procedures available as licenses for the various MAGNETOM systems: R.0 MAGNETOM
Licenses 1
H SVS
1
H CSI
31
P CSI
Harmony
+
-
-
Impact/Expert to Harmony Upgrade
+
-
-
Symphony
+
+
+
Vision to Symphony Upgrade
+
+
+
Sonata
+
+
+
Trio
+
-
-
Allegra
+
-
R.0 R.0
NOTE CSI measurements require the "Higher-Order Shim Channels" option to be installed on your MR system.
R.0
In addition, the 31P CSI measurement procedure requires the "Multi-Nucleus MRI & MRS" option. This option also includes the hardware requirements for measurements on 3He, 7Li, 13C, 19F, 23Na, 31P, and 129Xe.R.0
R.0
0.0
syngo MR 2002B Revision 04
iii
Licenses
MR Spectroscopy
0.0
iv
Operator Manual
PART
S
MR Spectroscopy
S.0
S.1 Basics The MRS Experiment... S.1–2 The Most Important Atomic Nuclei for MRS ... S.1–6 Chemical Shift ... S.1–8 Fine Splitting of the Resonance Lines via J Coupling ... S.1–11 Spectral Resolution ... S.1–14 The Relative Nuclear Ratio ... S.1–15 Quantification of the MRS Signals ... S.1–16 Signal-to-Noise Ratio (SNR) ... S.1–17 Nuclear Overhauser Effect (NOE) ... S.1–20 Decoupling ... S.1–25 Important MRS Signals ... S.1–26 The Most Important Signals of 1H MRS ... S.1–28 The Most Important Signals of 31P MRS ... S.1–31 From MRS Signal to Spectrum ... S.1–34 Characteristics of a Peak ... S.1–35
S.2 Techniques Single Volume Spectroscopy (SVS) ... S.2–2 Spin Echo Sequence ... S.2–3 STEAM Sequence ... S.2–4 Comparison of SE and STEAM Sequences ... S.2–5 Selecting the Echo Time for SVS Measurements ... S.2–6 Suppressing the Water Signal ... S.2–7
0.0
syngo MR 2002B Revision 04
S–1
Contents
MR Spectroscopy
Chemical Shift Imaging (CSI) ... S.2–8 Hybrid CSI Technique ... S.2–9 Localization Using Phase-Encoding... S.2–10 Signal-to-Noise Ratio with Phase-Encoding ... S.2–26 Chemical Shift Artifact during Hybrid CSI ... S.2–28
S.3 Applications Applications of the SVS Technique ... S.3–2 Applications of the CSI Technique ... S.3–3 1H Hybrid CSI Measurement of the Brain ... S.3–4 31P CSI Measurement of Muscle, Heart, and Liver ... S.3–8
S.4 Preparing the Measurement Positioning the Patient and Coil ... S.4–2 1H-MRS Measurements of the Brain ... S.4–3 31P-MRS Measurements of Muscles, Heart, or Liver ... S.4–6 Determining the Center of the Measurement Field ... S.4–13
S.5 Performing Measurements Transferring Protocols to the Program Control ... S.5–3 Reference Images ... S.5–6 Measuring Reference Images ... S.5–7 Loading Reference Images ... S.5–9 Editing the Display of Reference Images ... S.5–10 Determining the Nearest Image ... S.5–10
0.0
S–2
Operator Manual
MR Spectroscopy
Contents
Adapting the VOI for a SVS Measurement ... S.5–11 VOI Display ... S.5–12 Editing the VOI Graphically ... S.5–14 Editing the VOI Numerically ... S.5–20 Adapting a CSI Slice for a 2D CSI Measurement ... S.5–24 Display of a CSI Slice ... S.5–26 Using the Position and Orientation from a Reference Image ... S.5–30 Editing the CSI Slice Graphically ... S.5–31 Editing the CSI Slice and VOI Numerically ... S.5–33 Adapting the CSI Slab for a 3D CSI Measurement ... S.5–36 Display of a CSI Slab ... S.5–37 Editing the CSI Slab Graphically ... S.5–38 Editing the CSI Slab and VOI Numerically ... S.5–40 Saving a Reference Image with VOI and/or CSI Grid Definition ... S.5–43 Adapting the Parameters for a SVS Measurement ... S.5–44 Routine Parameter Card ... S.5–44 Contrast Parameter Card ... S.5–45 Resolution Common Parameter Card ... S.5–49 Sequence Common Parameter Card ... S.5–50 Adapting the Parameters for a CSI Measurement ... S.5–54 Routine Parameter Card ... S.5–54 Contrast Parameter Card ... S.5–55 Resolution Common Parameter Card ... S.5–56 Sequence Common Parameter Card ... S.5–63 Sequence Nuclei Parameter Card ... S.5–67 0.0
syngo MR 2002B Revision 04
S–3
Contents
MR Spectroscopy
Configuring Adjustments ... S.5–75 Shimming the Magnetic Field Automatically ... S.5–77 Water Suppression Adjustment... S.5–78 Setting the Adjustment Volume ... S.5–80 Automatic Frequency Adjustment ... S.5–81 Automatic Transmitter Adjustment ... S.5–82 Starting Scanning ... S.5–83 Monitoring the Raw Signal ... S.5–84 Saving Raw Data Automatically ... S.5–85 Editing Additional Protocols ... S.5–86 Copying Graphic Parameters ... S.5–87
S.6 Evaluating Data Automatically The Spectroscopy Task Card ... S.6–2 Calling up an Empty Spectroscopy Task Card... S.6–2 Loading Raw Data... S.6–3 Layout of the Spectroscopy Task Card ... S.6–7 Selecting the Display Mode... S.6–8 Loading Additional Raw Data Sets... S.6–10 Switching Data Sets ... S.6–11 Displaying the Spectrum ... S.6–13 Adapting the Signal Parameters ... S.6–14 Showing Peak Information ... S.6–20 Adding Comments to the Spectrum ... S.6–25 Saving and Documenting a Spectrum... S.6–27 Displaying Reference Images ... S.6–28 Loading Additional Reference Images ... S.6–29 Selecting Reference Images ... S.6–31 Image Display for the SVS Technique ... S.6–32 Image Display for the Hybrid CSI Technique ... S.6–33 0.0
S–4
Operator Manual
MR Spectroscopy
Contents
Modifying the Display of Images ... S.6–35 Saving and Documenting Reference Images ... S.6–41 Using Post-Processing Protocols... S.6–42 Selecting Another Post-Processing Protocol ... S.6–44 Transferring the Current Post-Processing Protocol ... S.6–46 Results Table ... S.6–48 Generating a Results Table ... S.6–49 Saving and Documenting the Results Table ... S.6–55 Evaluating Hybrid CSI Data Sets ... S.6–56 Evaluating Individual Voxels ... S.6–57 Evaluating All Voxels ... S.6–58 Creating a Spectral Map ... S.6–61 Creating Metabolite Images ... S.6–64 Editing the Display of a Spectral Map or Metabolite Image ... S.6–72 Saving and Documenting a Spectral Map or Metabolite Image ... S.6–75
S.7 Evaluating Data Interactively Editing and Applying Postprocessing Steps ... S.7–4 Applying Filters ... S.7–8 Applying Zero-Filling ... S.7–15 Water Reference Processing (Proton Spectroscopy only) ... S.7–17 Defining FID Shifting ... S.7–21 Eddy Current Compensation ... S.7–23 Applying Fourier Transform ... S.7–26 Correcting the Baseline ... S.7–28 Phase Correction ... S.7–35
0.0
syngo MR 2002B Revision 04
S–5
Contents
MR Spectroscopy
Frequency Shift ... S.7–43 Applying Curve Fitting ... S.7–45 Completing the Interactive Evaluation ... S.7–57 Arranging Postprocessing Steps ... S.7–58 Reopening Postprocessing Protocols ... S.7–60 Saving Protocol Changes ... S.7–61
S.8 Saving and Documenting Results Saving Results and Reference Images ... S.8–2 Loading Result and Reference Images into the Viewing Task Card ... S.8–5 Filming and Printing Results and Reference Images ... S.8–7 Closing the Data Set ... S.8–9 Closing Spectroscopy ... S.8–10
S.9 Editing Peaks and Peak Lists Editing Peaks ... S.9–2 Opening the Peak Editor ... S.9–3 Entering Peak Parameters ... S.9–5 Editing the Graphic Display of Peaks ... S.9–12 Completing the Peak Definition ... S.9–17 Adding a Peak ... S.9–18 Selecting a Peak from the List of Peak Templates ... S.9–18 Creating Peak Templates ... S.9–22
0.0
S–6
Operator Manual
MR Spectroscopy
Contents
S.10 Managing Post-Processing Protocols Opening a Protocol ... S.10–2 Editing a Protocol ... S.10–6 Changing the Number and Sequence of Postprocessing Steps ... S.10–6 Changing the Parameters of Postprocessing Steps ... S.10–11 Changing Protocol Properties ... S.10–12 Creating a New Protocol ... S.10–14 Saving the Protocol ... S.10–17 Deleting Protocols ... S.10–20 Exporting and Importing Protocols ... S.10–23
S.11 Performing Quality Assurance Quality Assurance for 1H-MRS ... S.11–2 Positioning the Phantom and Coil (1.0 and 1.5 T) ... S.11–2 Positioning the Phantom and Coil (3 T) ... S.11–3 Registering the Quality Measurement ... S.11–4 Selecting a QA Measurement Program ... S.11–5 Localizer Measurement ... S.11–6 Checking the Shim Quality ... S.11–6 Performing a Localized QA Measurement ... S.11–10 Checking the Results ... S.11–12
0.0
syngo MR 2002B Revision 04
S–7
Contents
MR Spectroscopy
Quality Assurance for 31P-MRS ... S.11–13 Positioning the Phantom and Coil ... S.11–13 Registering the Quality Measurement and Selecting a QA Measurement Program ... S.11–15 Localizer Measurement ... S.11–15 Checking the Shim Quality ... S.11–15 Performing the 31P-MRS QA Measurement ... S.11–16 Checking the Results ... S.11–17
S.12 Interactive Adjustment Automatic Adjustment with Interactive Control ... S.12–2 Interactive Adjustment ... S.12–5 Shimming the Magnetic Field Interactively... S.12–6 Manual Water Suppression ... S.12–15 Optimizing the Frequency ... S.12–23 Multinuclear Transmitter Adjustment... S.12–32
S.13 Protocols for MR Spectroscopy Protocols for In-vivo Application ... S.13–3 Protocols for Quality Assurance ... S.13–7 3D CSI Protocol ... S.13–9 Postprocessing Protocols ... S.13–10 Short Echo Times... S.13–11
S.14 Exporting Raw Data
0.0
S–8
Operator Manual
CHAPTER
S.1
Basics
S.1
MR Spectroscopy (MRS) determines the presence of certain chemical compounds selectively, non-invasively, and non destructively. This makes MR spectroscopy an important method for in-vitro- and in-vivo-examinations of the cell metabolism of tissue and organs. S.1 In healthy tissue, the metabolic products (metabolites) detected via MR spectroscopy are present in concentrations characteristic of specific tissues. Stress, functional disorders, or diseases can cause the metabolite concentrations to vary. MR spectroscopy enables you to observe such changes in concentration. S.1 However, the method has relatively low sensitivity and metabolite concentrations are low, generating signals typically 10000 times less intense than the water signal used in MR imaging. This makes it necessary to accumulate signals via repeated measurements, typically over several minutes. typically over several minutes. This limits the time resolution for detecting changes in concentration. S.1 For this reason, MR spectroscopy is primarily used to evaluate the status of cell metabolism. Additionally, this method allows you to monitor slow reactions for the effects of therapy over a period of time. S.1
0.0
syngo MR 2002B Revision 04
S.1–1
Basics
MR Spectroscopy
The MRS Experiment
S.1
As with MR imaging, MR spectroscopy is based on the magnetism of atomic nuclei. Certain atomic nuclei possess a magnetic moment. In quantum mechanics, the magnetic moment of the nucleus is termed "spin.” S.1 The magnetic moment is aligned when an external magnetic field is applied. Depending on the type of nucleus, the spins may be aligned in various discrete ways. For example, for protons there are only two types of alignment. Without the influence of additional forces, the spin prefers to align itself in field direction during the lower energy state. S.1 Since magnetic resonance techniques detect a large number of spins collectively (i.e. the nuclei of a macroscopic specimen, magnitude 1023), the individual alignments of the spins are never observed directly. S.1 The overall magnetization observed is a directional variable (vector) aligned parallel to the magnetic field without the influence of additional forces. In an MR experiment, the magnetization of the nuclei is deflected from this equilibrium. It is then oriented, for example, antiparallel (inverted) or perpendicular to the external magnetic field. It can continuously point in any intermediate direction as it returns to its equilibrium. It is described according to the Bloch equations and does not require quantum mechanics. S.1
0.0
S.1–2
Operator Manual
Basics
MR Spectroscopy
Electromagnetic waves are used to deflect the magnetization from equilibrium. The necessary energy or frequency of the waves is accurately defined precisely each nucleus; it is determined both by a property of the nucleus, the “gyromagnetic ratio”γ, and by the strength of the magnetic field applied B0: S.1 ω0 = γ Β 0
S.1
If this frequency is not fully obtained, no excitation is possible. The nuclei in an MR specimen have slightly different measurement frequencies 1) due to the inevitable inhomogeneity of the B0 field and 2) due to the "chemical shift" (see below). To excite as many of the nuclei of interest as possible, broadband excitation is selected using a short excitation pulse. The MR resonant frequencies are typically in the radio wave range. For example, the resonant frequency 63.6 MHz is obtained for protons at B0 = 1.5 S.1 Radiofrequency excitation aligns the nuclei such that there is a net magnetic moment perpendicular (transverse) to the main magnetic field. This moment precesses about the axis of the main field at the resonant (“Larmor”) frequency, generating a radiofrequency signal. The frequency is detected using an antenna ("coil”). S.1
0.0
syngo MR 2002B Revision 04
S.1–3
Basics
MR Spectroscopy
The alignment of these nuclei is degraded by random processes over a time period of a few to hundreds of milliseconds, leading to an exponential decay of the signal characterized by a relaxation time "T2*". At the same time, other random processes return the nuclei to their equilibrium alignment along the main magnetic field. This exponential process has a time constant of “T1*.” S.1 A spectroscopic MR experiment makes use of the fact that different chemical compounds cause a different degree of shielding of the main magnetic field at the location of the nucleus. Therefore, the resonance frequency for the precession of the same spins, e.g. protons, changes according to the chemical environment. → Page S.1–8, Chemical Shift S.1 This resonance frequency shift effect is termed "chemical shift.” The display of its frequency components is enabled via a Fourier transform of the time signal. This "NMR line spectrum" must therefore be interpreted as the concentrations of the spins with precession at a certain Larmor frequency versus the frequency shift. S.1
0.0
S.1–4
Operator Manual
MR Spectroscopy
Basics
In the most favorable case, unique assignment of a resonance line to a metabolite is possible. In unfavorable cases, the lines of several metabolites overlap or cover each other completely. Frequently, a metabolic product contains several nuclei detectable by MR with different resonance frequencies. As a result, this metabolic product generates a multi-line spectrum. S.1 A simple MRS experiment involves excitation of the spin, data recording, and conversion into a spectrum via Fourier transform. At the same time, a special requirement of a spectroscopy experiment becomes evident: especially high homogeneity of the B0 field over the specimen volume of interest is required to prevent line widening resulting from field inhomogeneities and to achieve optimal separation of the metabolite signals. S.1 For this reason, homogenization of the main magnetic field using relatively weak additional fields (called "shimming") is especially important in MRS experiments. S.1 The spectroscopy sequences described in this manual differ from the simple experiment provided here. The primary difference is that they additionally allow for localization of the measured area within the coil volume. S.1
0.0
syngo MR 2002B Revision 04
S.1–5
Basics
MR Spectroscopy
The Most Important Atomic Nuclei for MRS Magnetic Moment
S.1
S.1
Atomic nuclei that can be detected in MR experiments must possess a magnetic moment. The total moment of the nucleus consists of the moments of the constituent parts of the nucleus, the protons and neutrons. In a nucleus with an even number of protons and neutrons (i.e. an even atomic and mass number), the individual moments cancel out. Nuclei of this type cannot be detected by MR techniques. S.1 The magnitude of the magnetic moment is directly proportional to the gyromagnetic ratio which, in turn, defines the Larmor frequency at a certain magnetic field strength. S.1 The Larmor frequencies for 1.5 T are shown in the table below. As the Larmor frequencies change with the magnetic field strength, the frequency for other field strengths can be determined by performing a simple calculation. S.1
Spin quantum number
S.1
The setting options for the magnetic moment of the nucleus in the magnetic field and the number of possible energy states are characterized by the spin quantum number I. The value of I may be zero or a multiple of ½. The number of resulting energy states is (2I+1). S.1 The spin quantum number of hydrogen nuclei (protons) is I = ½ and therefore two energy states.
S.1
Nuclei with I = ½ result in narrow lines. Higher spin quantum numbers result in broader signals. S.1
0.0
S.1–6
Operator Manual
Basics
MR Spectroscopy
Sensitivity
S.1
An MRS experiment measures metabolite concentrations. The sensitivity of the measurement is determined by two factors: S.1 ❏ the natural isotopic abundance of the MR active nucleus ❏ a sensitivity specific to the nucleus determined by the magnetic moment (Here, specified relative to the sensitivity of protons.)
Table
S.1
S.1 S.1
Isotope
Spin quantum number I
Larmor frequency at 1.5 T [MHz]
Natural abundance [%]
Relative sensitivity [%]
H
1/2
63..6
99.9
100
19
F
1/2
59.8
100
84
31
P
1/2
25.7
100
6.6
Li
3/2
24.8
92.6
27
23
Na
3/2
16.8
100
10
13
C
1/2
16.0
1.1
1.6
1
7
0.0
syngo MR 2002B Revision 04
S.1–7
Basics
MR Spectroscopy
Chemical Shift
S.1
In different chemical compounds, the atomic nuclei are heavily shielded by the electron shell surrounding them. Because of the differing resonance frequencies, signals of identical nuclei (such as protons that are bonded to different elements or molecular components) therefore occur at different positions in the MR spectrum. In this way, it is possible to distinguish and identify molecular components as well as whole molecules or classes of substances. S.1
Quantitatively, the shift in resonant frequency caused by shielding σ as can be expressed as S.1 ω = (1 − σ) γ Β0
S.1
S.1
0.0
S.1–8
Operator Manual