Smiths Medical
Emergency and Transport Ventilation an introductory guide
Introductory Guide
15 Pages
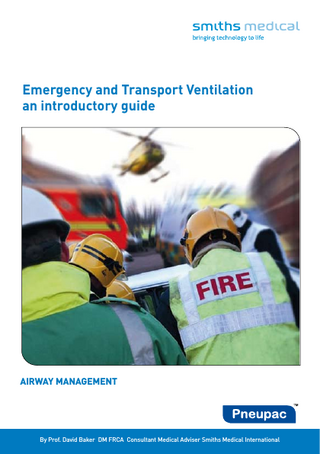
Preview
Page 1
Emergency and Transport Ventilation an introductory guide
AIRWAY MANAGEMENT
By Prof. David Baker DM FRCA Consultant Medical Adviser Smiths Medical International
Artificial ventilation is used in many different fields of medicine including emergency life support, anaesthesia and intensive care, and the transport of critically ill patients. In the ambulance, and other emergency services, ventilation is required as an essential response to a life-threatening event. Paramedical services are expected to provide a response at an advanced life support level. At this level ventilation is provided alongside the secure management of the airway and goes beyond simple techniques such as rescue breathing used in basic life support.
The lung: essential points of anatomy and physiology A working knowledge of the anatomy of the lungs and respiratory tract is essential for understanding the problems of emergency ventilation. Essentially the anatomy involves the airway (the structures which allow the passage of air from the mouth and nose to the lungs) and the lung tissue itself where gas exchange takes place.
transmit pressure to the stomach as well as the lungs. To avoid this, a good control of pressure and flow of gas is necessary since the protective muscle in the oesophagus (the oesophageal sphincter) begins to open above pressures of 20cm H2O.
Airway protection devices
JeeZg_Vl
This booklet is designed to introduce emergency medical personnel trained in advance life support to the use of portable gas–powered ventilators (PGPV) in prehospital medical care. It will hopefully be of use to paramedics, nursing and nonspecialised medical personnel alike both in and out of the hospital. Artificial ventilation is a subject that is sometimes not well understood outside the operating theatre and intensive care unit. The technical features of a PGPV may appear complicated and daunting compared with a more familiar device such as a self– reforming bag that has been widely used in emergency care.
1
Anatomy and physiology
This booklet is designed to
AdlZg_Vl
help you understand:
Anatomy and physiology
Introduction
Introduction
=VgYeVaViZ Hd[ieVaViZ 6YZcd^Yh IdcjZ GZigde]VgncZVa heVXZ :e^adii^h KdXVa[daY
A) Guedel pharyngeal airway
1
2
3
4
5
The essential anatomy and physiology of normal respiration and the processes involved in the provision of oxygen to the cells of the body. The mechanisms and assessment of respiratory failure in an emergency.
B) Laryngeal mask
The benefits and dangers of intermittent positive pressure ventilation outside the hospital. The function, checks, controls and monitoring of PGPV. The safe use of PGPV in emergency and transport ventilation.
C) Cuffed endotracheal tube
Airway Certain key points are important for establishing and continuing emergency ventilation. Perhaps the most important is that the upper part of the airway is shared with the pathway leading to the oesophagus. This means that mask ventilation will also
IgVX]ZV :hde]Vjh
At the level of the epiglottis the airway can be protected from the entry of foreign bodies and inhaled secretions. This is the basis of endotracheal intubation. Other techniques such as the laryngeal mask (LM) airway, form a protective seal above the level of the epiglottis. However the LM is not as secure as the endo-tracheal tube (ETT) and cannot be relied upon to withstand inflation pressures of greater than 25cm H2O. Outside the hospital, ventilation by oropharyngeal mask is used in emergency in conjunction with a Guedel airway to prevent obstruction of the pharynx by the tongue but the risk of inflation of the stomach is always present. The airway, which starts at the nasopharynx continues down into the lungs as the trachea, which divides at the carina and gives rise to the right and left main bronchi. After this there are continuing divisions of the bronchi leading to the alveoli.
1
Anatomy and physiology
1 Figure 2 - External and internal respiration
Figure 1 - General anatomy of the lungs
Anatomy and physiology
Anatomy and physiology
1
Atmospheric air Pco2 = 0.3mmHg
Po2 = 159mmHg Alveoli
Pco2= 40mmHg Po2= 105mmHg Pco2= 40mmHg Po2= 105mmHg
Pco2 = 45mmHg Po2 = 40mmHg
Pulmonary capillary Pco2= 40mmHg Po2= 100mmHg
To Lungs
External Respiration (a)
To left heart
To right heart
Internal Respiration (b)
To tissue cells
Breathing, ventilation and respiration
Pco2= 45mmHg Po2= 40mmHg
Pco2= 40mmHg Po2= 100mmHg
Systemic capillary
Interstitial fluid Po2= 40mmHg Pco2= 45mmHg Systemic tissue cells
The Lungs and their action The lungs are situated in each half of the thoracic cavity, separated by the mediastinum which contains the heart, the other major vessels and other structures, notably the oesophagus. Each lung cavity is called a hemithorax. The right and left lungs differ in the number of lobes, the right having three and the left, two lobes. During inspiration the action of the intercostals and diaphragm muscles produces an alteration in the shape of the thorax which creates a negative pressure inside the right
2
and left lungs and draws in air from the surrounding atmosphere. A good seal is obtained inside the thoracic cavity due to two lining membranes within the thorax, the visceral and parietal pleura. The space between these membranes is known as the pleural cavity and is normally empty except for a lubricating secretion which allows the pleura to slide easily over each other during normal respiration. In trauma the pleural cavities may fill with air (pneumothorax) or with blood (haemothorax).
Respiration is divided into external respiration, which describes the exchange of gases between the alveoli and blood and internal respiration which relates to the biochemical use of oxygen at the cellular level and the production of carbon dioxide. We have emphasised the importance of the seal in the chest cavity ensured by the pleura. When breathing takes place the volume of the thoracic cavity is increased and air is sucked in passively as a result of the negative pressure created. The changes in the lung volume occur as a result of diaphragmatic movement and alterations in the shape of the rib cage.
These terms are often used loosely to mean the same thing but they have specific meanings. Breathing may be defined strictly as the automatic mechanical process by which air passes in and out of the lungs to cause ventilation. Ventilation specifically means the movement of gas in and out of the areas of the lung where gas exchange is taking place. In practice this includes the alveoli and terminal bronchioles. The volume of the airway which does not take part in gas exchange is termed the anatomical dead space. Respiration is the term usually used in medicine to describe breathing and gas exchange combined. The overall purpose of respiration may be defined as oxygenation of the blood and removal of carbon dioxide.
3
Anatomy and physiology
1
Figure 3 - The negative pressure ventilation action of the thorax and diaphragm
Anatomy and physiology
Anatomy and physiology
1
Figure 4 - Lung volumes and capacities ml Maximum inspiration
6000
5000
Inspiratory reserve volume 3100 ml
4000
Vital capacity 4600 ml
Inspiratory capacity 3600 ml
Total
Lung
3000 Tidal volume 500 ml
Functional
Expiratory
2000
Reserve volume 1200 ml
Maximum expiration
Residual Capacity
1000
Residual volume 1200 ml
Capacity
6000 ml
2400 ml
Time
0
During diaphragmatic contraction the muscle flattens and thus increases the volume of the thoracic cavity. This action causes drawing in of air since the cavity is sealed by the pleural membranes. Diaphragmatic activity gives rise to about 75% of the air entry into the lungs. Alterations in the shape of the rib cage, which accompany the action of the diaphragm are produced by the intercostal muscles and pull the rib cage upwards and outwards. They cause a change in conformation of the rib cage which is rather like the handle of a bucket, causing a further increase in the volume of the cavity.
4
During expiration the volume of the thorax reverts to normal due to relaxation of the diaphragm into a dome shape. The internal intercostal muscles operate in the reverse direction to the externals, which caused the rib cage to open. These and the abdominal muscles tend to bring the ribs downwards and inwards causing a reduction in the size of the cavity. Normal ventilation depends essentially on the integrity of the pleural seal and the normal function of the muscles of respiration. If either fails (for example as a result of trauma causing pneumothorax and broken ribs) normal negative pressure ventilation will not result from breathing activity.
Spirometry Spirometry is the direct measurement of lung volumes from a subject breathing spontaneously. Figure 4 shows a normal spirogram where the average values of lung volumes are as follows: • Tidal volume (Vt): 500ml • Insipiratory reserve volume (IRV, the maximum extra inspiration that can be taken after a normal inspiration): 3100ml • Expiratory reserve volume (ERV, the maximum expiration possible after a normal expiration): 1200ml •R esidual lung volume (the gas remaining in the lung after a maximum voluntary exhalation): 1200ml Lung capacities are defined as sums of volumes. Thus: • Inspiratory capacity = IRV+Vt • Vital capacity= IRV+Vt+ERV • Functional residual capacity = ERV+RV • Total lung capacity = IRV+Vt+ERV+RV
In emergency and transport ventilation the tidal volume and functional residual capacity are the most important parameters listed here. Lung volumes in ventilation Tidal volume is the volume of gas moved in and out of the lungs during one breath. Normally in Intermittent Positive Pressure Ventilation (IPPV), the tidal volume, Vt, is calculated on the basis of 10ml/Kg. Patients who suffer from high risk of barotrauma due to damaged lungs are ventilated with a smaller Vt of 5 – 8ml/Kg. Neonates and infants with a small body mass are usually ventilated with a proportionally higher tidal volume of 12 – 15ml/Kg. Minute volume is the product of tidal volume and the frequency of ventilation. It is a parameter used in steady state ventilation of patients during anaesthesia and in the intensive care unit.
5
Anatomy and physiology
Control of breathing Normal breathing is controlled by a series of neurones sited in the hind part of the brain stem (the fourth ventricle). These nerve centres provide a cyclical discharge to ensure regular active inspiration (the Hering-Breuer reflex). The role of the medullary centre is to control the regular rhythm of breathing. It does this through sets of neurones which control both the inspiratory and expiratory timing.
Central control of breathing The neural control centres are: • The medullary centre, situated in the hind brain (in the floor of the 4th ventricle) • The pneumotaxic and apneustic centres which are situated in the mid brain (the pons varolii)
Figure 5 - Chemical Control of Breathing Breathing is also under the control of signals sent from special receptors (chemo receptors) which detect changes in CO2 and oxygen in the blood. There are both central and peripheral receptors. The central receptors respond to pH and CO2 changes in the blood and cerebrospinal fluid around the brain while the peripheral receptors respond to pH PCO2 and PO2 in the blood. The peripheral receptors are situated on the aortic arch and at the bifurcation of the carotid arteries. Carbon dioxide produces an increase in acidity due to a breakdown of carbonic acid into hydrogen and bicarbonate ions. In addition CO2 can easily pass across membranes including those of the blood-brain barrier. The peripheral chemo receptors are stimulated (1) by increase in CO2 and (2) by a fall in arterial pO2 from 100 to 50 mmHg (13.33 to 6.67 kPa). When stimulation occurs signals are sent to the respiratory centres causing the rate and depth of breathing to increase. Hyperventilation follows and this causes the elimination of CO2 until the blood CO2 and pH again become normal. Conversely, following hyperventilation, either voluntary or involuntary the blood CO2 levels are reduced and output from the chemo receptors stops, producing temporary apnoea.
6
1
The inspiratory centre begins to fire after three seconds of expiration during which it is inactive. While firing during two seconds of inspiration it activates both the diaphragm and the external intercostals muscles producing the expansion of the thoracic cage. At the end of this time it ceases to fire and the inspiratory muscles relax while expiration occurs passively as described above. However higher levels of the brain can activate the expiratory centres voluntarily if a forced expiration is required. The medullary centre is autonomous and its activity continued even if the higher centres of the brain are put out of action. The pneumotaxic centre in the pons is concerned with the co-ordination of inspiratory and expiratory activity, having an inhibitory effect on the inspiratory neurones. This means that it can regulate the degree of inspiration to prevent the lungs from over – distension. If the breathing rate rises the inspiratory time is shorter and the pneumotaxic centre is more active.
If the higher centres are removed or damaged, breathing continues in a rudimentary form in the form of gasping, from the apneustic centre (apneustic breathing). These control centres may be affected by trauma, raised intracranial pressure and toxic factors causing failure of the normal respiratory control mechanism.
Anatomy and physiology
Anatomy and physiology
1
The main conditions affecting the control of breathing are: • Trauma – increased cranial pressure following head injury • Infection - meningitis • Drugs • Poisons All these can lead to depression of ventilation and hypoxia
The apneustic centre in terms of evolution is the oldest and most basic of the respiratory control centres. Normally it provides stimulatory impulses to the inspiratory neurones and thus reduces expiration. It remains active when the pneumotaxic centre is not firing but is overridden by the higher centre. If the medullary and pneumotaxic centres are both non-functioning the apneustic centre provides a primitive, gasping form of basic respiration.
7
Anatomy and physiology Forces acting on the airways and the lungs A number of forces act against the entry of gas into the lungs, both during normal breathing and during artificial ventilation. In normal breathing the action of the chest and diaphragm causes a negative pressure in the thorax that sucks air in passively. Expiration is due to the elastic forces of the lung tissue. In artificial ventilation using positive pressure, work must be done by the ventilator to overcome the stiffness of the lungs and chest wall and also the resistance of the airways.
Characteristics of compliance Compliance is defined as: C=V/P where C is compliance, V is volume and P pressure. The total compliance of the lungs is a combination of the compliance of the lungs themselves and of the chest wall. The normal value for total static compliance is about 100 ml /cm H2O but in patients who are intubated and ventilated the value drops to 40 – 50 ml/cm H2O. Compliance may be decreased by lung inflammation, pulmonary oedema, pneumothorax, distension of the abdomen, ARDS (Adult Respiratory Distress Syndrome), fibrosis, thoracic abnormalities and by airway obstruction. Conversely, compliance may be increased by distension of the alveoli, destruction of the septa (as in emphysema) and by loss of the lung elastic tissue.
8
2
Respiratory failure
Compliance The term compliance refers to the ease of expansion of the lungs and the walls of the thorax. A high compliance means that expansion is easy whereas low compliance means the opposite. Low compliance occurs when the alveoli are damaged, filled with fluid or there is reduced surfactant (a fluid secreted in the normal lung which lowers surface tension and stops the alveoli from collapsing). It also occurs when the muscles of respiration are damaged. Resistance Resistance or more correctly airway resistance is the term used to describe the resistance to gas flow through the airways and the endotracheal tube. The origin of resistance is based on the friction of the gas molecules moving through the airways. Paradoxically, most resistance occurs in the wider bore upper airways since, although the cross sectional area of the lower airways is smaller there are many more of them and so the overall cross sectional area available for gas conduction is higher. Resistance is therefore increased in pathology that affects the smaller airways such as bronchospasm in asthma, and oedema of the mucosal lining of the bronchi. In addition, intubation increases the overall resistance to flow as does turbulent flow where the gas is not flowing in smooth layers into the lung.
The failure of the normal process of breathing and the flow of gas in and out of the lungs is generally termed respiratory failure. The failure of ventilation leads to a build up of CO2 in the alveoli and a reduction in the level of oxygen. The relationship is given by the alveolar air equation shown in table 1.
The reduction of oxygen partial pressure in the alveoli causes less oxygen to be delivered to the erythrocytes and ultimately to the mitochondria. There is thus a failure of the normal cellular respiratory process which generates energy in the form of ATP (Adenosine Triphosphate). Although the cells can move over to a different form of biochemical energy generation called anaerobic metabolism, this is much less efficient in generating ATP and can only proceed at the cost of producing lactic acid, which is ultimately detrimental to the function of the body cells.
Respiratory failure
Anatomy and physiology
1
Table 1 - Basic equations governing ventilation of lungs The concentration of oxygen in the alveoli in contact with the blood is given by the alveolar air equation:
PaO2 = PiO2 – Pa CO2 / R
Where Pa O2 and Pa CO2 are the partial pressures of oxygen and carbon dioxide in the alveoli, and PiO2 is the partial pressure of oxygen in the inspired gas. R is the respiratory quotient which has normal value of 0.8 The level of CO2 is itself proportional to the amount of gas passing in and out of the alveoli (alveolar ventilation) according to the following equation:
.
. . PeCO2 = VCO2 x k / Va
.
Where VCO2 is the amount of carbon dioxide released into the alveoli per minute, Va is alveolar ventilation and k is a constant. If the CO2 could be removed from the expired gas by absorption onto soda lime (as in the case with certain types of anaesthesia) hypoxia does not occur provided the minimum oxygen requirement is provided. However this is not possible in emergency ventilation and so an adequate level of ventilation must be maintained to remove the CO2 produced.
9
Respiratory failure
Respiratory failure The causes of respiratory failure are: Central: failure of the control system, head injury, intoxication, drug – induced, infection, stroke, cardiac arrest a
b Peripheral: failure of the mechanical system of breathing trauma causing pneumothorax and lung collapse with loss of the surrounding vacuum when the chest expands, pain on breathing causing an attenuation of the thoracic and diaphragmatic function, fatigue of the breathing system due to chronic respiratory disease, failure of oxygen transfer due to pulmonary oedema
10
The failure of ventilation of the lungs leads to a failure of delivery of oxygen to the body cells. ‘Respiratory failure’ is therefore strictly a cascade involving a failure of breathing, ventilation, external and internal respiration. It should be noted that damage leading to failure of delivery of oxygen to the mitochondria can occur at any point of the linked processes. Clinically, respiratory failure may be acute, chronic or acute on chronic. Respiratory failure – partial and total The term ‘respiratory failure’ is normally used when breathing fails either partially or completely. Respiratory failure may occur suddenly or gradually. Cardiac arrest for example causes an abrupt cessation of the flow of blood to the brain including the neural control centres for respiration. This in turn causes an abrupt arrest of normal breathing giving the classic picture of the cardiac arrest patient with no pulse or respiration. Respiratory arrest may also be gradual as a result of the mechanisms listed above. In this situation there is a gradual onset of hypoxia which, in the extreme situation will cause the heart to stop. This is known as secondary cardiac arrest and is the most common situation in children.
2 3
Assessment of respiratory failure in emergency: - how to decide when to ventilate the patient Assessment of respiration is a fundamental part of the primary and secondary survey (Airway, Breathing and Circulation) in emergency management.
Respiratory failure
Respiratory failure
2
Table 2 - The Glasgow Coma Score Eye Opening (E) 4 = Spontaneous 3 = To voice 2 = To pain 1 = None
Verbal Response (V) 5 = Normal conversation 4 = Disoriented conversation 3 = Words, but not coherent 2 = No words... only sounds 1 = None
Motor Response (M) 6 = Normal 5 = Localizes to pain 4 = Withdraws to pain 3 = Decorticate posture 2 = Decerebrate 1 = None
Total = E+V+M If breathing is assessed as inadequate or absent intermittent positive pressure ventilation must be started. If there is a GCS score < 9 the patient should be intubated and ventilated
Assessment of respiration starts by establishing the conscious level of the patient using the Glasgow Coma Scale (GCS) as shown in table 2. In acute failure there is often no time to use the Glasgow scale and the quicker AVPU system is used (to assess if the patient is Alert, or responds to Voice commands, Painful stimuli or is Unresponsive).
If the patient is conscious and having difficulties in breathing he may experience symptoms such as dyspnoea. Dyspnoea is a sensation of shortness of breath or ‘difficulty in breathing’ or tightness in the chest. These symptoms may be accompanied by physical signs of abnormal breathing - see table 3.
11
Assessment of respiratory failure in emergency:
Table 3 - Physical signs of abnormal breathing If the patient is unconscious then rapid assessment of both the airway and breathing is necessary using the patient’s physical signs alone. This is done by looking, listening and feeling the patient. Looking Inspection of the patient will show the rate of respiration, its regularity and depth. It will also show whether the respiratory pattern is normal. It is important to be able to recognise normal respiration. Looking at the patient will also reveal signs of reduced oxygenation of the blood as cyanosis of the lips and mucous membranes (the colour of the skin is far less reliable). Inspection will therefore (1) show whether he is breathing or not and (2) if breathing, whether the rate is normal (>8<20 per minute at rest for a normal adult), (3) whether the respiration is of normal depth and has the correct form. In abnormal respiration and in airway blockage the chest and abdominal movements work against each other in a see-saw movement.
Intermittent Positive Pressure Ventilation Emergency and transport ventilation IPPV is required: a. to support total respiratory failure. b. to provide emergency ventilation as part of cardio – pulmonary life support (Airway, Breathing and Circulation). c. during transport of the patient in respiratory failure from an emergency site to the hospital. d. during transport of a ventilator – dependent patient from hospital to hospital. e. IPPV will also be required when the breathing rate and /or tidal volume are inadequate and to support the patient’s own respiratory efforts (partial respiratory failure).
3 3
Intermittent Positive Pressure Ventilation
Respiratory failure
2
Respiratory failure
Listening It is not necessary to use a stethoscope to gather valuable information about respiration. The UKRC guidelines for basic life support emphasise the importance of simply listening for sounds of respiration at the patient’s mouth to determine whether air is going in and out. The stethoscope has little place in the rapid initial survey of breathing failure. It is of considerable use later in checking that an adequate airway has been established, for example following intubation. Feeling (palpation) Finally, placing the hands upon the patient’s chest and feeling the movements is also valuable in assessing reduction in respiration. Experience gathered with normally breathing persons is valuable in being able to produce a quick assessment where breathing is threatened.
12
13
Intermittent Positive Pressure Ventilation Figure 6 - The Pneupac VR1 ventilatory resuscitator
Benefits of IPPV The main benefits of IPPV are:
Emergency and transport ventilation Artificial ventilation of the lungs takes place outside the hospital in several different settings. Broadly these may be divided in to emergency and transport ventilation. Although these are often combined (as in the US where both are classed as transport ventilation) they have different objectives and protocols and require different equipment. It is convenient therefore to view pre-hospital ventilation as (1) Emergency ventilation, which may be sub-divided into resuscitation ventilation and ventilation for less acute emergencies and (2) Transport ventilation, which involves the movement of a critically - ill but stable patient who is on a ventilator from one location to another, either inter- or intra-hospital.
14
3
The Pneupac Parapac ventilator
Intermittent Positive Pressure Ventilation (IPPV) is required when normal breathing is inadequate or absent. IPPV replaces the normal cycle of breathing with the important difference that gas enters the lungs as a result of positive pressure drive rather than being sucked in as in case of normal breathing.
1
Reversal of respiratory failure and hypoxia by re-establishing ventilation.
2
ontrol of respiration where pathology exists and reduction of collateral damage in C situations such as trauma.
3
Ability to control the alveolar architecture. In acute respiratory failure there may be collapse of parts of the lung. IPPV opens up the lungs and keeps the alveoli open, causing a reduction in physiological dead-space.
4
Control of CO2 and therefore blood pH levels in developing respiratory acidosis.
5
Allows the administration of major pain relief in trauma (which would otherwise stop or seriously reduce spontaneous breathing).
Problems of IPPV 1) Hyperventilation The term hyperventilation strictly means providing ventilation to the alveoli that is greater than the patient’s normal physiological requirements in a resting condition. In other words, the minute volume is too high. All normal people hyperventilate at some time for example during exercise when greater quantities of CO2 are produced by the body and have to be eliminated. Hyperventilation during IPPV carries the principle risk that the CO2 level in the alveoli is lowered leading in turn to reduced levels of CO2 in the blood causing respiratory alkalosis. The main problem associated with this condition is that the reduction in the level of acidity in the blood (a decrease in pH level) reduces the efficiency of the haemoglobin to release oxygen. Lowered CO2 levels also cause constriction of the
Intermittent Positive Pressure Ventilation
Intermittent Positive Pressure Ventilation
3 3
blood vessels in the brain and reduction in oxygen levels. In some circumstances high minute volume is permitted when there is raised intracranial pressure following head injury but this is not a technique to be used by non-medical personnel in the pre hospital situation.
15
Intermittent Positive Pressure Ventilation 2) Barotrauma Over-ventilation may produce a high inflation pressure as a result of setting too high a tidal volume. In the normal physiological situation the interior of the alveoli are not subjected to high pressures at all since the lungs function by drawing air in passively following the creation of a negative pressure inside the thoracic cavity. When high inflation pressures are applied to this fragile tissue the lung may be over inflated. If there are weak points in the alveoli air may break the walls and escape into the thoracic cavity (pneumothorax). This situation is called barotrauma. High inflation pressures and barotraumas can result from tidal volumes that are too high or from constriction of the airways due to emergencies such as asthma or as a result of exposure to toxic fumes. Prevention of barotrauma is achieved by (a) correct setting of the ventilator and (b) by automatic release of the over-inflation by blow-off valve or pressure relief valve.
3) Hypoventilation Under-ventilation of the lungs leads to increased CO2 levels, acidosis and hypoxia as a result of the ventilation and alveolar air equations given in table 1.
Hypoventilation may be: 1
2
eliberate as a result of d incorrect settings of tidal or minute volume
The function of the Portable gas-powered ventilator Portable Gas-Powered Ventilators (PGPV) date from the early 1970s. Up to that point emergency IPPV could only be provided in an emergency using bag – valve devices which had themselves only been in use since the 1950s following the invention of the self-reforming bag by Henning Ruben. The essential idea behind the PGPV was to have a ventilator that was totally portable together with its gas supply, that could provide ventilation that did not have major peaks in the peak inspiratory pressure and which had a controlled flow of gas to the patient.
43
ccidental as a result of a disconnection of IPPV or leaks in the circuit.
Hypoventilation cannot lead to immediate physical damage to the lungs (as is the case in barotrauma) but over a period of time may lead to collapse of parts of the lung (atelectasis) and has serious consequences due to the build-up of CO2 and hypoxia in the lungs. Effects on circulation and the reduction of venous return IPPV causes a reduction in the venous blood return to the heart and reduces cardiac output, since the heart pumps according to the amount of blood flowing into it (Starling’s Law of the Heart). To minimize the cardiac effects the ratio of the time for inspiration to expiration (the I:E ratio) must be kept at 1:2 and tidal volumes should be kept to a minimum.
16
Portable Gas-Powered Ventilators
Portable Gas Powered Ventilators
Intermittent Positive Pressure Ventilation
3
P200D - Parapac
Pressure and flow generation Early versions of the PGPV were pressure generators. This means that the ventilator controls pressure such that the pressure waveform remains unchanged with changes in respiratory system mechanics, but volume and flow vary. The problems with this approach is that if the lungs are stiff (low compliance) or there was significant resistance in the airways a set pressure limit could be reached without passing an adequate tidal volume in the lungs. Some modern PGPV still operate this way but most are time–cycled, volume
preset flow generators. In this mode of operation the ventilator controls flow and the flow and volume waveforms remain unchanged with changes in respiratory mechanics, but pressure varies. Usually a constant flow of gas (usually less than 40 litres/minute) is generated by the ventilator over a preset time period delivering a set tidal volume. Pressure generation is used in emergency for ventilation of neonates and infants who have fragile lungs.
17
Portable Gas Powered Ventilators Figure 7 - Inside a Parapac Ventilator
The function of the PGPV
Variable relief valve
Demand valve
Demand detector
Design features How do PGPV work? The heart of a PGPV is a pneumatic oscillator which provides the timing of the ventilation by the build-up of pressure of gas flowing to one side of a spring assisted valve. The operation of the oscillator can be controlled by two needle valves which can control both the frequency and tidal volume of the delivered ventilation. The PGPV is powered by a standard compressed oxygen cylinder at 150 bar (about 2000psi). This pressure is lowered through a reducing valve down to 30 - 90psi. The driving pressure is not critical because when the gas enters the ventilator there is a built in regulator capable of operating over this pressure range which accurately regulates the final pressure. Inside the ventilator the final gas pressure is accurately controlled to a maximum of 40cm H2O or 60cm H2O with safety valves ensuring against too high a ventilation pressure being delivered to the patient.
18
4 3
Portable Gas Powered Ventilators
Portable Gas Powered Ventilators
3 4
PGPV should: be light, rugged and simple to operate 2 be capable of operation in all common environmental conditions 3 f unction as constant flow generator 4 have an optimum I:E ratio to allow sufficient time for thoracic compression in CPR 5 be able to entrain air into the delivered gas mixture (air mix) and therefore be capable of delivering either 100% or 45 – 50% oxygen. 6 be capable of adult and child operation (but small children require a pressure generator) 7 be fitted with a demand valve system 8 have minimal gas consumption for driving the ventilator 9 be capable of operating in a magnetic resonance imaging unit (MRI compatible) 1
Flow block
Input/output block
Regulator
Air mixing The first PGPV could only deliver 100% oxygen (or air if this were used as the driving gas). 100% oxygen is appropriate for emergency ventilation but is not indicated for prolonged periods of ventilation. In 1985, PGPV were fitted with air-entrainment valves. This is a venturi device that entrains air which is mixed with the driving gas being delivered to the patient. The resulting mixture is between 45 and 55% depending on the model. One major benefit of the entrainment valve is that the life of the cylinder of compressed oxygen driving the ventilator is prolonged.
In addition, PGPV should have the following safety features: 1 2 3
4 5
pressure limitation
cycling indicator
isconnection and high d pressure alarms
patient inflation manometer
driving gas low alarm
19
Portable Gas Powered Ventilators
5 3
Figure 8 - How a PGPV operates
18
6
3
How to use a PGPV for emergency ventilation
19
20
Inflation Pressure Monitor
Frequency Control
Main Pneumatic Switch (CMV/DemandDemand Switch)
This section describes how to ventilate a patient using a PGPV. The Parapac is
12
used as a teaching example since it is widely used in paramedical emergency practice
4 1
5
13
Oscillator
15
10 To Patient Valve
2 14 17
11
9 Demand Detector
Demand Valve
7 8
Variable Relief Valve
16 Air-mix Switch
The principle of operation of a switch (16) is open and the driving gas Parapac has only two controls for Parapac ventilator is shown in figure 8. passes through the needle valve (14) frequency and tidal volume. Inside Compressed oxygen (1) is fed to the main and into the entrainment part (15). This the ventilator the frequency control is pneumatic switch (3). When this is open valve is adjusted so that its flow always connected to needle valve 6 while the gas is fed to a pressure regulator unit (4) matches the entrainment requirements tidal volume control is connected to which ensures that the gas delivered to of the mixing device and in this way needle valves 13 and 14 to control the the ventilator is at constant pressure of 100% driving gas is fed to the patient. flow to the patient. 2.7 atmospheres (about 38psi). If airmix is selected the ventilator The inflation pressure is limited by The gas is then fed to the oscillator delivers 45% oxygen to the patient by the spring-loaded variable relief valve (5) which is a device that switches the sending gas through needle (13) to the (11). During expiration the gas flow flow of gas to the patient on and off at nozzle of the entrainment device (15). is vented to the atmosphere at 20 a frequency controlled by the precision This draws in ambient air through part through the normally-open valve (18). needle valve (6) feeding two coupled flow 19 via the non-return valve (17) . The If the supply gas fails this also allows control needles (13 and 14). If the Parapac resulting gas mixture passes to the the patient to breathe air from the is set to deliver 100% (no ‘air mix’) the patient via the output connection (10). surrounding atmosphere.
20
Demand valve Through the 1990s there were further developments that improved the operation and flexibility of PGPV. The most important of these was the development of the demand valve. Prior to the introduction of this device PGPV could only ventilate patients who were in complete respiratory failure. Unlike the bigger ventilators available in hospital they could not respond to patients who still had some respiratory efforts. If the patient was still breathing but the efforts were inadequate (as in head injury) it was necessary to remove
The ventilator should be connected to the oxygen supply and the controls set to CMV/demand, the ventilation frequency at 12 per minute, the delivered oxygen concentration to 100% and the relief pressure (if variable) to 40cm H2O. The tidal or minute volume control should be set to an average value, depending on the size of the patient being ventilated. When the controls are set the ventilator will cycle and the low pressure alarm will sound until connected to the patient.
Figure 9 - Essential steps in ventilating Looking around Parapac: the controls, monitors and other features The essential steps in ventilating a patient using a PGPV are as follows: Alarm Module Adjustable pressure
the respiratory effort using an opiate drug. The demand valve made it possible for the ventilator to respond to patients own respiratory efforts. In a PGPV fitted with a demand valve if the patient takes an adequate breath the automatic operation of the ventilator is suppressed and ventilation with 100% oxygen is ensured by the patient’s own efforts. If the respiration is inadequate however, the ventilator takes over the controlled ventilation once more.
The patient should then be placed in the correct ventilation position (usually on his back) and the airway held open by tilting the head and lifting the chin. Learning this position is part of training in providing life support which is essential before using a PGPV. The mask must be connected securely to the patient hose from the ventilator and applied to the patient’s mouth and nose using two hands to maintain a good seal, at the same time ensuring that the airway remains open. If the mask seal is correct then ventilation of the patient’s lungs will begin. When possible, it is advised to lower the high pressure alarm level to just above the actual ventilation pressure to make it work most effectively.
Portable Gas Powered Ventilators
Portable Gas Powered Ventilators
4
Inflation pressure monitor Frequency control
Low gas supply indicator
CMV Demand
Airmix
Tidal volume control
21
Portable Gas Powered Ventilators Figure 10 - Clinical monitoring during ventilation
Monitoring IPPV on a PGPV
Check: 1
To ensure that IPPV is effective: Look at the chest and ensure that it rises and falls with the audible operation of the ventilator. If the PGPV you are using has a manometer the reading during the inspiratory phase of ventilation should be between 10 and 15cm H2O. Listen to the sounds made by the ventilator and ensure that no alarms are sounding. If an alarm is sounding the reason may be that the pressure being delivered to the patient is too high (due to airway blockage but sometimes due to kinking of the delivery tube or blockage of the patient’s airways due to bad positioning, a foreign body or constriction of the bronchial tubes (as in asthma).
22
5 3
the rise and fall of the chest with operation of the ventilator.
2
the patient’s colour (from the mucous membranes).
3
the oxygen saturation (pulse oximetry) – this should be above 95% when ventilated with 100% oxygen. Alternatively the airway pressure may be too low, due to a major leak in the circuit, a poor mask seal, or a too low tidal volume. The correct action is to adjust the mask seal or ensure that the patient circuit is correctly connected. CMV/Demand ventilation If the patient is partially conscious and is still making breathing efforts the PGPV should be set to CMV/Demand. Explain what you are going to do to the patient and place the mask from the patient circuit over the patient’s face ensuring a correct seal. If the patient is breathing adequately the demand valve on the ventilator will provide an oxygen flow and respiratory support during the inspiratory phase. In the DEMAND mode the ventilator does not provide any respiratory support. When the patient breathes spontaneously all work has to be done by the patient. The automatic nature of this function does not remove the need for close examination of the patient’s colour and conscious level.
Apart from clinical observation of the patient the monitoring system of the PGPV provides valuable information. The following notes relate to the Parapac:
Figure 11 - Alarm module
Portable Gas Powered Ventilators
Portable Gas Powered Ventilators
5
The normal ventilation cycling light which indicates that the pressure delivered to the patient is passing through 10cm H2O. The high pressure alarm: this is both an audible and visual alarm which indicates that the pressure delivered to the patient is above that set by the operator or the manufacturer (if a fixed relief valve is fitted) Normal high values are 40cm H2O but in the US 60cm H2O is more common. 40cm H2O is safer if a mask is being used for ventilation. The high pressure alarm will sound if the airway is blocked at any level (either inside or outside the patient) from kinking of the circuit or constriction of the medium and small bronchi. The alarm will also sound if the ability of the alveoli to expand properly is reduced (decreased compliance). (See Note at the end of this section).
The low pressure alarm: this sounds and flashes if the inflation pressure fails to pass through 10cm H2O and indicates that there is either a disconnection of the ventilator or a serious leak of gas in the patient circuit.
23
Portable Gas Powered Ventilators
5 3
Conclusions Transport Ventilation Although emergency and transport ventilation are terms that are often interchanged they have quite specific meanings. Emergency ventilation is required to overcome life threatening hypoxia whereas transport ventilation relates to any situation where a patient who is stable and requires ventilation has to be moved. Such a move may be inside the hospital (for example from the ER to the ward), from the ward to an imaging facility or from hospital to hospital by road and/or air. Transport ventilation is normally under direct medical control but a ventilator such as Parapac may be used safely for the task. Movement of a ventilated patient is associated with a number of clinical hazards and the following rules for transport ventilation must be observed.
• the operation of the ventilator should be checked before connection to the patient • sufficient bottled oxygen must be available for the duration of the transport • an alternative means of ventilation such as a bag valve device must always be with the patient • the airway must be definitive and secured (tracheal intubation is the norm) • airway positioning must be checked by auscultation every time the patient is moved • the ventilator settings and alarms must be checked every time the patient is moved • the patient must be clinically observed at all times • no patient on a ventilator must ever be left unattended
Note: The blow-off valve cuts-off the inspiratory time whereas the pressure relief is only a pressure limitation system. During partial occlusion or just
This booklet has provided a basic introduction to: 1 The essential anatomy and physiology of normal respiration: the processes involved in the provision of oxygen to the cells of the body. 2 The mechanisms and assessment of respiratory failure in emergency. 3 The benefits and dangers of intermittent positive pressure ventilation outside the hospital. 4 The function, checks, controls and monitoring of PGPV. 5 The safe use of PGPV in emergency and transport ventilation. It does not replace the need to read carefully the instruction manual for any ventilator you may be using nor for the need to train in the correct use of this equipment.
It is not necessary to have a detailed understanding of the engineering and function of the PGPV to be able to use them safely but an understanding of the controls and monitoring devices is of the utmost importance. No amount of safety and monitoring devices can ever replace the need for continuing clinical observation of the patient who is being ventilated. No patient on a ventilator should ever be left unattended and an alternative means of ventilation (such as a bag – valve device) should always be at hand.
Portable Gas Powered Ventilators
Portable Gas Powered Ventilators
5
The PGPV is a valuable tool in emergency and critical care management. I hope that this booklet will help you to feel comfortable in their use in your everyday practice.
slightly higher resistance/pressure the system does not stop the inspiratory phase but continues but at a safe (adjusted) level.
24
25
THE DETAILS GIVEN IN THIS LEAFLET ARE CORRECT AT THE TIME OF GOING TO PRESS. THE COMPANY RESERVES THE RIGHT TO IMPROVE THE EQUIPMENT SHOWN
Smiths Medical International Ltd Hythe, Kent CT21 6JL UK Tel: +44 (0)1303 260551 Fax: +44 (0)1303 266761 www.smiths-medical.com Smiths Medical, part of the global technology business Smiths Group Pneupac, Pneupac VR1, Portex and the Smiths Medical Design Mark are trademarks of the Smiths Medical family of companies. © 2006 Smiths Medical family of companies. All rights reserved. Literature No. LIT/AV2591